Research objectives
Our main objective is to study Canadian Arctic gossans as an analogue to ancient hydrothermal systems on the planet Mars. The gossans are characterized by ochre-coloured surface deposits that are quite easily recognized using satellite images (figure 1). However, the composition of these gossans can vary at local scale (one meter or less), which requires remote sensing instruments closer to the outcrop to determine its mineralogical composition. The precise elemental composition and the potential for preservation of the biosignature must be obtained through the analysis of the samples: this is an information that cannot be obtained remotely.
We therefore propose to study the composition of the gossans at the spatial scales of the orbit, the rover and the sample (figure 2). From this, the specific objectives presented under figure 2 are defined, which have been chosen with the aim of realizing a methodology similar to the one used during the Martian missions.
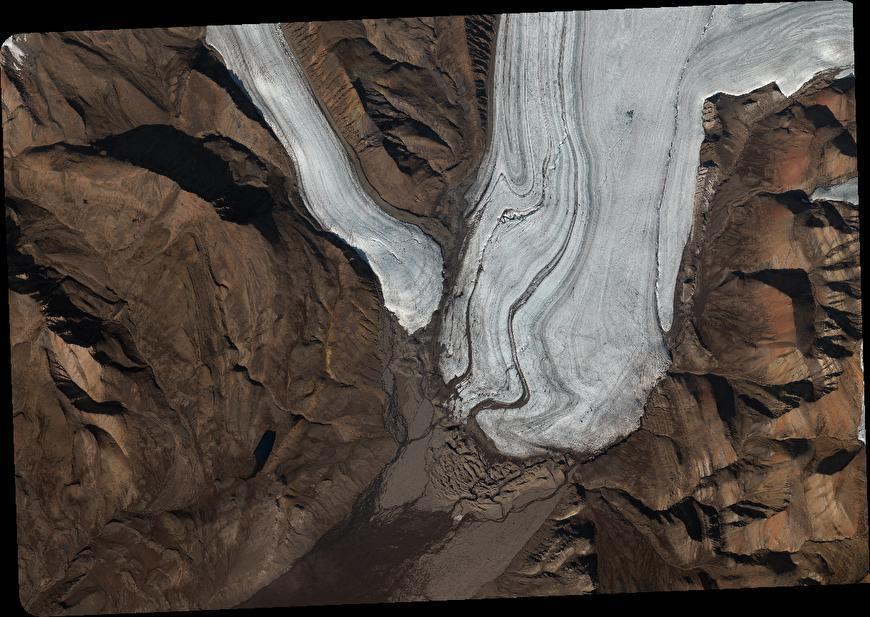
Figure 1 : Gossans near the MARS station, Axel-Heiberg Island, Nunavut. Image captured by the WorldView satellite (Copyright 2019 DigitalGlobe Incorporated).
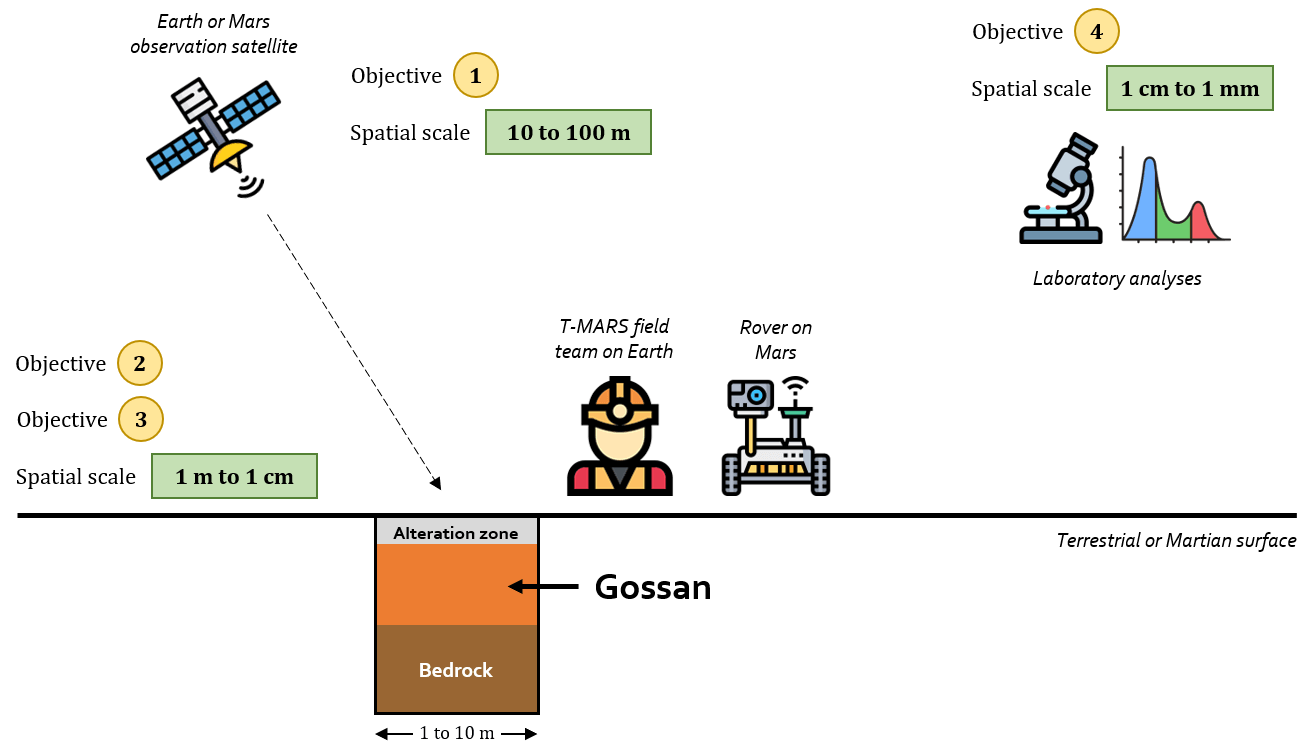
Figure 2 : Research objectives and their respective spatial scales.
Gossans have been detected at various locations in the Canadian Arctic and some have already been studied in the field. Our study area is located near the McGill Arctic Research Station (MARS - not to be confused with the title of our research project T-MARS, Terrestrial Mineral Analysis by Remote Sensing), on Axel Heiberg Island in Nunavut. This region was found to be particularly rich in gossans and evaporite domes, which is consistent with the presence of a paleo-hydrothermal system [1,2,3].
The first step of our project is to establish the regional context and remote predictive mapping [4] of the gossans on Axel Heiberg Island, at a spatial scale of about ten to a hundred meters. These maps will be useful in preparation for the field campains and the work that will be carried out on site [4]. The geoscientific data used for the mapping include:
- geological maps provided by the Geological Survey of Canada;
- topographic maps;
- satellite images from sensors such as Landsat, Spot, Ikonos and WorldView;
- the Arctic Digital Elevation Model (ArcticDEM).
In our case, we will produce a predictive map of the gossans on Axel Heiberg Island to determine their abundance, which ones are accessible for field sampling and which seem to be the most interesting scientifically, based on their regional context and composition. The process of remote predictive mapping is analogous to the use of data acquired by remote sensing instruments in orbit around the planet Mars, in order to determine the most promising landing site for a lander or a rover [5].
The second step of our project is to investigate the composition of gossans horizontally, at a spatial scale of meter to centimeter. The research will be conducted on Axel Heiberg Island during two filed campaigns in the summers of 2022 and 2023 in order to visit as many outcrops as possible. This step is analogous to the scientific operations that would be carried out by a rover or a drone on Mars.
Acquire localized remote sensing data
- Measured parameter: localized reflectance of gossans
- Measuring instrument: portable spectroradiometer
- Spatial scale of measurement: centimetric
- Utility: to establish the mineralogical composition at the surface at different locations on the gossans and to validate remote sensing observations acquired at coarser resolutions
Acquire surficial samples
- Measured parameter: collection of samples for further laboratory analysis
- Measuring instrument: shovels, picks, plastic bags
- Spatial scale of measurement: centimetric
- Utility: determine the mineralogical and chemical composition of iron caps
The third step of our project is to investigate the composition of the gossans vertically, at a spatial scale of meter to centimeter. The research that will be conducted on the field at Axel Heiberg Island is analogous to the scientific operations that could be conducted by a Martian rover using a core drill and remote sensing instruments. Past exploration of the Canadian Arctic gossans on Victoria Island and Axel Heiberg Island has revealed that the gossans are stratified, but that the stratification of the different gossans varies [1,6]. A closer investigation of the stratigraphy will increase the knowledge of the vertical composition of gossans and their formation and evolution processes. It will also allow us to target the specific layers that most probably host life (and potentially have hosted life on Mars in the past).
Acquire multispectral images of a cross-sectional view of gossans
We will then dig 30-50 cm deep trenches in the selected gossans to obtain a cross-sectional view. This process is analogous to a rover acquiring a coring sample (for example, the corer of the next ExoMars 2020 mission of the European Space Agency is expected to reach depths of at most two meters). Images of these sections will be acquired to determine the stratigraphy of the outcrop. This information will allow to distinguish and categorize the different layers. Samples will be acquired for each identified layer, using organically sterile material to avoid contamination. The samples will be brought back to the laboratory for further analysis.
The fourth step of our project is to investigate the spectral signature, the composition and the biosignature content of the reported samples. The analyses will be conducted in different laboratories by the students, under the supervision of the researchers. Bringing back to the laboratories samples similar to those collected on Mars for further study is in line with the objectives of the Mars 2020 rover, for which the samples will be acquired, sealed, stored on board and then deposited on the surface of Mars to be retrieved by a later mission.
Investigate the spectral signature of samples
We will characterize the spectral reflectance of each sample in the spectroscopy laboratory of Dr. Lemelin at the Université de Sherbrooke. The reflectance spectra will be acquired using a portable spectroradiometer and following the specifications of the Canadian Space Agency's (CSA) planetary analogue material suite [7], which details standardized parameters for measurements. The most relevant spectra will then be shared with the CSA's planetary analogue materials suite under the Mars analogue section [7].
Investigate the composition of samples
We will characterize the composition of the samples using X-ray fluorescence (XRF) and X-ray diffraction (XRD) instruments at the Facility for Electron Microscopy Research at McGill University, under the supervision of astrobiologist Dr. Léveillé. FRX instruments provide information on the elemental and chemical composition of the samples, while the DRX provides information on the mineralogy of the material.
Investigate the biosignature of samples
The biosignature content of the samples will be analyzed at McGill University under the supervision of astrobiologist Dr. Léveillé. More specifically, we will target the elemental and textural signatures of jarosite and associated minerals. While the preservation of organic matter is expected to be low on Mars, minerals may retain information for much longer periods of time as they are not subjected as much to oxidative processes and radiation effects as organic matter [8,9]. For these reasons, minerals related to hydrothermal systems and alteration minerals are considered as primary exploration targets on Mars [10,11].
Jarosite and related iron sulphate minerals are generally produced from low-temperature aqueous alteration in sulfide-rich hydrothermal systems [12,13]. Thus, they are of particular interest on Mars and in our study region. It has recently been shown that jarosite formed without the presence of life has spectral differences from jarosite formed in the presence of life [14]. It has also been shown that the study of terrestrial occurrences of jarosite can help to reinterpret the history of the presence of water on Mars, on the basis of data collected by rovers. The conclusion is that the presence of jarosite, rather than suggesting acidic and water-limited environments, suggests much more habitable conditions at the beginning of Mars [15].
Hydrothermal systems on Earth are well known to contain diverse microbial communities, many of which leave characteristic biosignatures in rocks and minerals, including mineralized filamentous forms [12,13]. The advantage of investigating samples in the laboratory is that a high level of detail can be obtained regarding the interactions between microbes, organic matter and mineral matter. In fact, the study of samples collected from Mars may be the only way to conclusively detect the past presence of life.
Our approach can be used to investigate biosignatures and develop an unambiguous set of them. In addition to simulating the analysis of samples from the planet Mars, this effort will also help to better understand the preservation of biosignature in Mars-like minerals over geological time, in cold and arid climates. We will combine LIBS and Raman spectroscopy in the laboratory to characterize samples and identify biosignatures. These will be further investigated using a scanning and transmission electron microscope at the Facility for Electron Microscopy Research at McGill University.
References
Percival and Williamson (2017) Morphology, mineralogy and geochemistry of gossans, Axel Heiberg Island, Nunavut, in Williamson, M.-C. (2017) GEM 2 High Arctic Large Igneous Province (HALIP) activity: workshop report; Geological Survey of Canada, Open File 8151, 60 p.
Harrington et al. (2019) A Polarimetric SAR and Multispectral Remote Sensing Approach for Mapping Salt Diapirs: Axel Heiberg Island, NU, Canada, Canadian Journal of Remote Sensing, 45(1), 54-72.
Zentilli et al. (2019) Paleo-Hydrothermal Predecessor to Perennial Spring Activity in Thick Permafrost in the Canadian High Arctic, and Its Relation to Deep Salt Structures: Expedition Fiord, Axel Heiberg Island, Nunavut, Geofluids, 2019(9502904), 1-33.
Harris et al. (2012) Remote Predictive Mapping: An Approach for the Geological Mapping of Canada’s Arctic, Dr. Imran Ahmad Dar (Ed.), ISBN: 978-953-307-861-8, InTech.
Rogers and Bandfield (2009) Mineralogical Characterization of Mars Science Laboratory Candidate Landing Sites from THEMIS and TES Data, Icarus 203(2), 437-453.
Percival and Williamson (2016) Mineralogy and spectral signature of reactive gossans, Victoria Island, NT, Canada, Applied Clay Science, 119, 431–440.
Cloutis et al. (2015) The Canadian space agency planetary analogue materials suite, Planetary and Space Science, 119, 155-172.
Banfield et al. (2001). Mineralogical biosignatures and the search for life on Mars. Astrobiology, 1(4), 447-465.
Pavlov et al. (2012). Degradation of the organic molecules in the shallow subsurface of Mars due to irradiation by cosmic rays. Geophysical research letters, 39(13).
Varnes et al. (2003). Biological potential of Martian hydrothermal systems. Astrobiology, 3(2), 407-414.
National Academies of Sciences, Engineering, and Medicine (2019) An Astrobiology Strategy for the Search for Life in the Universe. Washington, DC: The National Academies Press.
Izawa et al. (2011) Weathering of post-impact hydrothermal deposits from the Haughton impact structure: implications for microbial colonization and biosignature preservation. Astrobiology, 11(6), 537-550.
Williams et al. (2015). Preserved filamentous microbial biosignatures in the Brick Flat gossan, Iron Mountain, California. Astrobiology, 15(8), 637-668.
Loiselle et al. (2018) A spectral comparison of jarosites using techniques relevant to the robotic exploration of biosignatures on Mars, Life, 8(4), 61.
Potter-McIntyre and McCollom (2018) Jarosite and Alunite in Ancient Terrestrial Sedimentary Rocks: Reinterpreting Martian Depositional and Diagenetic Environmental Conditions, Life, 8(3), 32.